IEEE TRANSACTIONS ON ANTENNAS AND PROPAGATION, VOL. 60, NO. 12, DECEMBER 2012
6023
[11] R. Liu, A. Degrion, J. J. Mock, and D. R. Smith, “Negative index material composed of electric and magnetic resonators,” Appl. Phys. Lett., vol. 90, no. 26, Jun. 2007. [12] Z. Szabó, G.-H. Park, R. Hedge, and E.-P. Li, “A unique extraction of metamaterial parameters based on Kramers-Kronig relationship,” IEEE Trans. Microwave Theory Tech., vol. 58, no. 10, pp. 2646–2653, Oct. 2010. [13] V. Lucarini, J. J. Saarinen, K. E. Peiponen, and E. M. Vartiainen, Kramers-Kronig Relations in Optical Materials Research. Berlin, Germany: Springer-Verlag, 2005.
Substrate Integrated Waveguide Cavity-Backed Wide Slot Antenna for 60-GHz Bands Ke Gong, Zhi Ning Chen, Xianming Qing, Peng Chen, and Wei Hong
Abstract—A wide slot antenna backed by a substrate integrated waveguide (SIW) cavity is presented and investigated for the enhancement of operating bandwidth at 60-GHz band. The 10-dB return loss impedance bandwidth of the antenna increases from 3% to 11.6% with consistent radiation performance as the width to length ratio (WLR) of the rectangular slot cutting from the SIW increases from 0.12 to 0.71. Its dual-resonance 4 anoperation mechanism is verified by a parametric study. Three 2 tenna arrays with different slot WLRs are designed and fabricated with a printed circuit board (PCB) process at the 60-GHz bands. The measured results show that the antenna array with slot WLR of 0.71 achieves the gain of 10–12 dBi and the cross-polarization levels of better than 25 dB in both - and -planes over the enhanced operating bandwidth of about 11.6%. Index Terms—Cavity-backed antenna, dual-resonance, slot antenna, substrate integrated waveguide (SIW), 60-GHz band.
I. INTRODUCTION Waveguide slot array antennas and cavity-backed slot antennas are widely used in radar and communication systems. The slot with a large width-to-length ratio has been successfully employed to enhance the operating bandwidth of the antennas [1]–[4]. On the other hand, substrate integrated waveguide (SIW) slot array antennas and cavity-backed slot antennas have been extremely investigated in recent years. However, the reduced waveguide height of the SIW greatly Manuscript received March 31, 2012; revised June 06, 2012; accepted July 30, 2012. Date of publication August 14, 2012; date of current version November 29, 2012. This work was supported in part by the National 973 project of China 2010CB327400, the National Nature Science Foundation of China (NSFC) under Grant 60921063, Research Fund for the Doctoral Program of Higher Education of China under Grant 20100092120013 and in part by the Agency for Science, Technology and Research (A*STAR), Singapore, Terahertz Science & Technology Inter-RI Program under Grant #0821410040 by the Institute for Infocomm Research, Agency for Science, Technology and Research (A*STAR), Singapore. K. Gong, P. Chen and W. Hong are with the State Key Laboratory of Millimeter Waves, Southeast University, Nanjing 210096, China (e-mail: [email protected]; [email protected]; [email protected]). Z. N. Chen is with Department of Electrical and Computer Engineering, National University of Singapore, Singapore 117583 and also with the Institute for Infocomm Research, Agency for Science, Technology and Research (A*STAR), 138632, Singapore (e-mail: [email protected]; [email protected]). X. Qing is with the Institute for Infocomm Research, Agency for Science, Technology and Research (A*STAR), 138632, Singapore (e-mail: [email protected]). Color versions of one or more of the figures in this communication are available online at http://ieeexplore.ieee.org. Digital Object Identifier 10.1109/TAP.2012.2213060
Fig. 1. The SIW cavity-backed wide slot antenna with slot
WLR = 0 4. :
narrows the impedance bandwidth of the antennas to around 3% or less [5]–[8]. In this communication, a planar wide slot antenna backed by an SIW cavity is proposed for bandwidth enhancement at 60-GHz bands. The impedance bandwidth, radiation patterns and gain of the antenna are analyzed against the WLR of the slot. In the proposed antenna, the backed cavity is not only a reflector for the radiating slot, but also a radiation element for the whole antenna. To verify the dual-resonance operating mechanism of the antenna, a parametric study is carried out using CST. The cavity-backed slot antennas show compact size, which are suitable for array application. With the optimized elements, three 2 2 4 antenna arrays with different WLRs are prototyped using PCB technology at 60 GHz bands. II. SIW CAVITY-BACKED WIDE SLOT ANTENNA Fig. 1 depicts the geometry of the proposed SIW cavity-backed wide slot antenna. A transverse wide slot with the WLR of 0.4 ( s s = 0 4) is etched onto the broad-wall of an SIW. The SIW is short-circuited at a distance 0 from the center of the slot. An inductive window is inserted into the SIW at a distance 1 from the short-circuited end of SIW, where 1 is about twice of 0 . Thus, an SIW cavity-backed wide slot antenna is formed with size of siw1 and 1 , and it is fed by the SIW with the width of siw . 1 and are the diameter of the via-hole and the spacing between adjacent via-holes, respectively. The slotted-cavity and the feeding SIW are formed with the conditions of 0 5 and 1 0 0 1 [9]. 1 In the proposed antenna, the slot operates at its dominant mode with the overall length of approximate a half operating wavelength, and the backed slotted-cavity resonates at its dominant mode frequency. The antenna is fabricated onto a piece of a 0.635-mm thick RO3006 substrate with a dielectric constant of r = 6 15 and loss tangent of tan = 0 0025, and simulated and optimized using CST. The proposed cavity-backed slot antenna exhibits a dual-resonant characteristic, and its operating bandwidth can be enhanced by increasing the slot WLR. Fig. 2 shows the simulated return losses of the antennas with the WLRs of 0.2, 0.4, 0.6 and 0.71, respectively. During the WLR varies from 0.2 to 0.71, the good impedance matching with two resonances is maintained by tuning the parameters including w , and nearly four times enhancement in s, 1, siw1 , 0 and
:
d
:
D =P 000e
W =L
d
d d W W D P
D =0015
:
'
:
L d W
d
d
W
:
6024
IEEE TRANSACTIONS ON ANTENNAS AND PROPAGATION, VOL. 60, NO. 12, DECEMBER 2012
WLR = 0 2 W = W = 1:85 L = 1:33 W = 0:925 d = 0:665 d = 1:25 d = 1:375 WLR = 0:4 W = 1:85 W = 2 L = 1:43 W = 0:99 d = 0:69 d = 1:3 d = 1:35 WLR = 0:6 W = 1:85 W = 2:05 L = 1:55 W = 1 d = 0:755 d = 1:45 d = 1:25 WLR = 0:71 W = 1:85 W = 1:95 L = 1:65 W = 1:13 d = 0:735 d = 1:47 d = 1:24 D = 0:2 P = 0:4 W = 6 L = 10
Fig. 2. The simulated return loss of the SIW cavity-backed slot antennas with : : , , different slot WLRs. ( , , ; : , , , , , , , ; , , , , : , , ; , , : , , , , ; and , , , all in mm.) ,
Fig. 5. The simulated return loss of the antenna with different lengths of the slotted-cavity.
Fig. 6. The simulated return loss of the antenna with different widths of the slotted-cavity.
E (y 0 z)
H (x 0 z)
Fig. 3. The simulated radiation patterns of the proposed antennas at 60 GHz and (b) -planes. with different WLRs in (a)
Fig. 7. The simulated return loss of the antenna with different slot lengths.
III. ANTENNA CHARACTERISTICS AND PARAMETRIC STUDY Fig. 4. The simulated gain of the proposed antennas with different slot WLRs.
WLR = 0 71
bandwidth is obtained. For the : , the top side of the slotted-cavity is nearly open with the wide slot, and the 10-dB return loss bandwidth reaches up to 11.6% (57–64 GHz). Fig. 3 presents the simulated radiation patterns of the cavity-backed slot antennas at 60 GHz with the WLRs of 0.2, 0.4, 0.6 and 0.71, respectively. It can be seen that the antennas keep consistent radiation patterns as varying the WLRs, and the higher gain is obtained for the bigger WLR under the same ground plane size of 10 mm 2 6 mm, as shown in Fig. 4.
The proposed cavity-backed slot antenna features the enhanced operating bandwidth by increasing the slot WLR where two resonances are well excited simultaneously when the slot WLR varies from 0.12 to 0.71 with consistent radiation performances. One of the resonances is generated by the radiating slot, where the SIW cavity functions as a reflector. The other one resonance is the resonant dominant mode of the slotted-cavity, where the slotted-cavity acts as a radiation element. Therefore, the slotted-cavity acts as not only a reflector but also a radiating element. In order to verify the mechanism of the dual-resonance behavior, a parametric study is carried out using the commercial software package, CST. The antenna with the WLR of 0.4 is discussed, and its parameters,
IEEE TRANSACTIONS ON ANTENNAS AND PROPAGATION, VOL. 60, NO. 12, DECEMBER 2012
WLR = 0 5
6025
WLR = 0 71
Fig. 10. The photograph of the fabricated 2 : and (c) : . (b)
2 4 arrays. (a) WLR = 0 12, :
Fig. 8. The simulated radiation patterns of the cavity-backed wide slot antenna with slot WLR of 0.71 at two resonant frequencies.
X = Y = 0:45 X = 0:5 = 1:8 L = 8 L = 2:15 L = 3:4
Fig. 11. (a) The simulated return loss and (b) the measured return loss of the , , arrays with the waveguide-to-SIW transition. ( , , , , , , , , , all in mm.).
Y = 0:4 d = 0:53 S = S = S W = 2:8 L = 1:9 W = 1:5
C. Length of the Transverse Slot Fig. 7 shows the variation of the return loss for different slot length
Ls while the WLR is fixed at 0.4. The lower resonance frequency shifts
down but the upper one maintains almost unchanged as the slot length is increased from 1.42 mm to 1.48 mm. These results indicate that the lower resonant frequency is mainly controlled by the slot length. On the other hand, the bandwidth is increased with the increased slot length.
Fig. 9. The geometry of the 2
2 4 antenna array prototypes.
unless otherwise stated, are fixed as Ls = 1:44 mm, d0 = 0:665 mm, d1 = 1:25 mm, Ww = 0:81 mm, and Wsiw1 = 2:2 mm. A. Length of the Slotted-Cavity Fig. 5 shows the effect of the length of the slotted-cavity, Wsiw1 on the impedance matching of the antenna. As Wsiw1 is varied from 1.92 mm to 2.07 mm, the upper resonant frequency shifts down and the lower one hardly changed leading to a narrower bandwidth. These results suggest that the upper resonance frequency can be controlled by adjusting the length of the slotted-cavity. B. Width of the Slotted-Cavity Fig. 6 shows the simulated return loss against the width of the slottedcavity. As the width, d1 , is varied from 1.24 mm to 1.27 mm, the upper resonance shifts down while the lower resonance is hardly changed. Moreover, it can be found that the 10-dB return loss impedance bandwidth of the antenna decreases from 6.5% for d1 = 1:24 mm to 5% for d1 = 1:27 mm with increasing d1 .
D. Discussion The parametric study reveals that the lower resonance results from the slot resonance by the wave transmission through the inductive window, and the upper resonance is a resonant mode of the slotted-cavity which is controlled by d1 and Wsiw1 . The radiation performances of the radiating slot and that of the slotted-cavity in the proposed antenna are expected to be consistent against the varying WLR of the slot. Fig. 8 shows the simulated radiation patterns of the cavity-backed wide slot antenna with the slot WLR of 0.71 at its two resonant frequencies. It is observed that the radiation patterns are very similar at the two different resonances. This attributes to the fact that the etched area on the broad-wall of the slotted-cavity can be modeled as a magnetic wall with the high-permittivity substrate [10], and similar field distributions in the slot and the magnetic wall will be excited by the feeding SIW with a TE10 mode. The simulated gain at the lower and upper resonance is 7.4 dBi @ 59.2 GHz and 7.9 dBi @ 62.8 GHz, respectively. As a result, the impedance and radiation bandwidths of the cavity-backed slot antenna can be enhanced by increasing the slot WLR. IV. ARRAY EXAMPLES WITH DIFFERENT WLRS OF SLOT Owning to the substrate in SIW, the proposed antennas feature compact size suitable for array application. In this section, three SIW
6026
IEEE TRANSACTIONS ON ANTENNAS AND PROPAGATION, VOL. 60, NO. 12, DECEMBER 2012
Fig. 12 shows measured gain of the antenna arrays. There is about 1-dB drop in the measured gains compared with the simulated ones, which may be caused by the dielectric loss of the substrate. The fluctuation of the measured gain of the three arrays is less than 2 dB over their operating bandwidth. The three arrays exhibit similar radiation patterns. Fig. 13 shows the measured normalized radiation patterns of the array with the WLR = 0:5. The 3-dB beamwidths in the E (y 0 z)- and H (x 0 z)-planes are 48000e and 18000e , respectively. Furthermore, the cross-polarization levels of less than 025 dB are observed in both the E - and H -planes. Fig. 12. (a) The simulated gain and (b) the measured gain of the arrays with different WLRs.
V. CONCLUSIONS Planar bandwidth enhanced wide slot antennas backed by an SIW cavity have been proposed and investigated at 60-GHz bands, where the backed cavity has played the role of not only a reflector but also a radiating element. By increasing the WLR of the transverse slot from 0.12 to 0.71, the impedance bandwidth has been enhanced from 3% to 11.6% with the introduction of dual-resonance operation. Over the enhanced bandwidth, the consistent radiation patterns have been achieved. The parametric study has been carried out to verify the dual-resonance behavior of the antenna. For comparison, three cavity-backed slot antenna arrays with the different WLRs have been designed and experimentally validated at 60-GHz bands using a standard PCB process.
Fig. 13. The simulated and measured normalized radiation patterns of the 2 4 array with the WLR of 0.5. (a) E (y z )-plane and (b) H (x z )-plane.
0
0
2
cavity-backed slot antenna arrays with the different WLRs are designed and prototyped on the RO3006 substrate using PCB technology at 60 GHz bands. Fig. 9 shows the geometry of these 2 2 4 antenna arrays, in which the feeding SIW is modified with the inductive via-hole. A compact eight-way tree-shape power divider splits the power into eight ways equally and excites all the elements in-phase. The antenna arrays are excited by a WR-15 waveguide through a stepped waveguide-to-SIW transition [11]. Fig. 10 shows the photographs of the prototypes with the WLRs of 0.12, 0.5 and 0.71, respectively. For the array with the WLR of 0.12, the distances between the adjacent elements are Lx = 3:3 mm (0:6600150 ) and Ly = 3:15 mm (0:6300150 ) along the x and y axis, respectively, where 00150 is the wavelength at 60 GHz in free space. For the arrays of WLR = 0:5 and 0.71, the distances are Lx = 4 mm (0:800150 ), Ly = 3:15 mm (0:6300150) and Lx = 3:45 mm (0:6900150), Ly = 3:37 mm (0:67400150), respectively. Fig. 11 shows the simulated and measured return loss of the antenna arrays with the waveguide-to-SIW transition. The measured return losses follow the trend of the simulated ones well with a slight frequency shifting which may be caused by the fabrication tolerance and the permittivity fluctuation. For the arrays of WLR = 0:12 and 0.5, the measured impedance bandwidths are 3.3% and 6.5%, respectively, which are consistent with the simulated results. For the array with WLR = 0:71, the simulated impedance bandwidth reaches up to 11.5%, whereas the measured return loss exhibits a slight deterioration, namely 9 dB at 57.3 GHz and 8.2 dB at 59.2 GHz, respectively. The array with the larger WLR is more sensitive to the fabrication tolerance, because the slot with a bigger WLR is closer to the inductive window and the via-holes. In practical applications, the antenna can be directly integrated into circuits, and it can be expected that the impedance matching and reliability of the antenna without any transition would be improved.
ACKNOWLEDGMENT The authors would like to thank J. Xu, Y. Zhang, W. Liu, T. S. Ping See, and Z. Kuai for their valuable discussions and kind help in fabrication and measurement.
REFERENCES [1] X. Yu, D. Ni, S. Liu, Z. Li, and W. Wang, “Design of a wideband waveguide slot array antenna and its decoupling method for synthetic aperture radar,” in Proc. Eur. Microwave Conf., 2008, pp. 135–138. [2] W. Hong, N. Behdad, and K. Sarabandi, “Size reduction of cavitybacked slot antennas,” IEEE Trans. Antennas Propag., vol. 54, no. 5, pp. 1461–1466, May 2006. [3] J. Hirokawa, H. Arai, and N. Goto, “Cavity-backed wide slot antenna,” IEE Proc., vol. 136, no. 1, pp. 29–33, Feb. 1989. [4] Y. Miura, J. Hirokawa, M. Ando, Y. Shibuya, and G. Yoshida, “Double-layer full-corporate-feed hollow-waveguide slot array antenna in the 60 GHz-band,” IEEE Trans. Antennas Propag., vol. 59, no. 8, pp. 2488–2851, Aug. 2011. [5] G. Q. Luo, Z. F. Hu, L. X. Dong, and L. L. Sun, “Planar slot antenna backed by substrate integrated waveguide cavity,” IEEE Antennas Wireless Propag. Lett., vol. 7, pp. 236–239, 2008. [6] J. C. Bohorquez, H. A. F. Pedraza, I. C. H. Pinzon, J. A. Castiblanco, N. Pena, and H. F. Guarnizo, “Planar substrate integrated waveguide cavity-backed antenna,” IEEE Antennas Wireless Propag. Lett., vol. 8, pp. 1139–1142, 2009. [7] G. Q. Luo, Z. F. Hu, Y. Liang, L. Y. Yu, and L. L. Sun, “Development of low profile cavity backed crossed slot antennas for planar integration,” IEEE Trans. Antennas Propag., vol. 57, no. 10, pp. 2972–2979, Oct. 2009. [8] X. Chen, K. Wu, L. Han, and F. He, “Low-cost high gain planar antenna array for 60-GHz band applications,” IEEE Trans. Antennas Propag., vol. 58, no. 6, pp. 2126–2129, June 2010. [9] F. Xu and K. Wu, “Guided-wave and leakage characteristics of substrate integrated waveguide,” IEEE Trans. Microw. Theory Tech., vol. 53, no. 1, pp. 66–73, Jan. 2005. [10] A. S. Al-Zoubi, A. A. Kishk, and A. W. Glisson, “A linear rectangular dielectric resonator antenna array fed by dielectric image guide with low cross polarization,” IEEE Trans. Antennas Propag., vol. 58, no. 3, pp. 697–705, Mar. 2010. [11] T. Kai, J. Hirokawa, and M. Ando, “A stepped post-wall waveguide with aperture interface to standard waveguide,” in Proc. Antennas and Propagation Soc. Int. Symp., 2004, vol. 2, pp. 1527–1530.
6023
[11] R. Liu, A. Degrion, J. J. Mock, and D. R. Smith, “Negative index material composed of electric and magnetic resonators,” Appl. Phys. Lett., vol. 90, no. 26, Jun. 2007. [12] Z. Szabó, G.-H. Park, R. Hedge, and E.-P. Li, “A unique extraction of metamaterial parameters based on Kramers-Kronig relationship,” IEEE Trans. Microwave Theory Tech., vol. 58, no. 10, pp. 2646–2653, Oct. 2010. [13] V. Lucarini, J. J. Saarinen, K. E. Peiponen, and E. M. Vartiainen, Kramers-Kronig Relations in Optical Materials Research. Berlin, Germany: Springer-Verlag, 2005.
Substrate Integrated Waveguide Cavity-Backed Wide Slot Antenna for 60-GHz Bands Ke Gong, Zhi Ning Chen, Xianming Qing, Peng Chen, and Wei Hong
Abstract—A wide slot antenna backed by a substrate integrated waveguide (SIW) cavity is presented and investigated for the enhancement of operating bandwidth at 60-GHz band. The 10-dB return loss impedance bandwidth of the antenna increases from 3% to 11.6% with consistent radiation performance as the width to length ratio (WLR) of the rectangular slot cutting from the SIW increases from 0.12 to 0.71. Its dual-resonance 4 anoperation mechanism is verified by a parametric study. Three 2 tenna arrays with different slot WLRs are designed and fabricated with a printed circuit board (PCB) process at the 60-GHz bands. The measured results show that the antenna array with slot WLR of 0.71 achieves the gain of 10–12 dBi and the cross-polarization levels of better than 25 dB in both - and -planes over the enhanced operating bandwidth of about 11.6%. Index Terms—Cavity-backed antenna, dual-resonance, slot antenna, substrate integrated waveguide (SIW), 60-GHz band.
I. INTRODUCTION Waveguide slot array antennas and cavity-backed slot antennas are widely used in radar and communication systems. The slot with a large width-to-length ratio has been successfully employed to enhance the operating bandwidth of the antennas [1]–[4]. On the other hand, substrate integrated waveguide (SIW) slot array antennas and cavity-backed slot antennas have been extremely investigated in recent years. However, the reduced waveguide height of the SIW greatly Manuscript received March 31, 2012; revised June 06, 2012; accepted July 30, 2012. Date of publication August 14, 2012; date of current version November 29, 2012. This work was supported in part by the National 973 project of China 2010CB327400, the National Nature Science Foundation of China (NSFC) under Grant 60921063, Research Fund for the Doctoral Program of Higher Education of China under Grant 20100092120013 and in part by the Agency for Science, Technology and Research (A*STAR), Singapore, Terahertz Science & Technology Inter-RI Program under Grant #0821410040 by the Institute for Infocomm Research, Agency for Science, Technology and Research (A*STAR), Singapore. K. Gong, P. Chen and W. Hong are with the State Key Laboratory of Millimeter Waves, Southeast University, Nanjing 210096, China (e-mail: [email protected]; [email protected]; [email protected]). Z. N. Chen is with Department of Electrical and Computer Engineering, National University of Singapore, Singapore 117583 and also with the Institute for Infocomm Research, Agency for Science, Technology and Research (A*STAR), 138632, Singapore (e-mail: [email protected]; [email protected]). X. Qing is with the Institute for Infocomm Research, Agency for Science, Technology and Research (A*STAR), 138632, Singapore (e-mail: [email protected]). Color versions of one or more of the figures in this communication are available online at http://ieeexplore.ieee.org. Digital Object Identifier 10.1109/TAP.2012.2213060
Fig. 1. The SIW cavity-backed wide slot antenna with slot
WLR = 0 4. :
narrows the impedance bandwidth of the antennas to around 3% or less [5]–[8]. In this communication, a planar wide slot antenna backed by an SIW cavity is proposed for bandwidth enhancement at 60-GHz bands. The impedance bandwidth, radiation patterns and gain of the antenna are analyzed against the WLR of the slot. In the proposed antenna, the backed cavity is not only a reflector for the radiating slot, but also a radiation element for the whole antenna. To verify the dual-resonance operating mechanism of the antenna, a parametric study is carried out using CST. The cavity-backed slot antennas show compact size, which are suitable for array application. With the optimized elements, three 2 2 4 antenna arrays with different WLRs are prototyped using PCB technology at 60 GHz bands. II. SIW CAVITY-BACKED WIDE SLOT ANTENNA Fig. 1 depicts the geometry of the proposed SIW cavity-backed wide slot antenna. A transverse wide slot with the WLR of 0.4 ( s s = 0 4) is etched onto the broad-wall of an SIW. The SIW is short-circuited at a distance 0 from the center of the slot. An inductive window is inserted into the SIW at a distance 1 from the short-circuited end of SIW, where 1 is about twice of 0 . Thus, an SIW cavity-backed wide slot antenna is formed with size of siw1 and 1 , and it is fed by the SIW with the width of siw . 1 and are the diameter of the via-hole and the spacing between adjacent via-holes, respectively. The slotted-cavity and the feeding SIW are formed with the conditions of 0 5 and 1 0 0 1 [9]. 1 In the proposed antenna, the slot operates at its dominant mode with the overall length of approximate a half operating wavelength, and the backed slotted-cavity resonates at its dominant mode frequency. The antenna is fabricated onto a piece of a 0.635-mm thick RO3006 substrate with a dielectric constant of r = 6 15 and loss tangent of tan = 0 0025, and simulated and optimized using CST. The proposed cavity-backed slot antenna exhibits a dual-resonant characteristic, and its operating bandwidth can be enhanced by increasing the slot WLR. Fig. 2 shows the simulated return losses of the antennas with the WLRs of 0.2, 0.4, 0.6 and 0.71, respectively. During the WLR varies from 0.2 to 0.71, the good impedance matching with two resonances is maintained by tuning the parameters including w , and nearly four times enhancement in s, 1, siw1 , 0 and
:
d
:
D =P 000e
W =L
d
d d W W D P
D =0015
:
'
:
L d W
d
d
W
:
6024
IEEE TRANSACTIONS ON ANTENNAS AND PROPAGATION, VOL. 60, NO. 12, DECEMBER 2012
WLR = 0 2 W = W = 1:85 L = 1:33 W = 0:925 d = 0:665 d = 1:25 d = 1:375 WLR = 0:4 W = 1:85 W = 2 L = 1:43 W = 0:99 d = 0:69 d = 1:3 d = 1:35 WLR = 0:6 W = 1:85 W = 2:05 L = 1:55 W = 1 d = 0:755 d = 1:45 d = 1:25 WLR = 0:71 W = 1:85 W = 1:95 L = 1:65 W = 1:13 d = 0:735 d = 1:47 d = 1:24 D = 0:2 P = 0:4 W = 6 L = 10
Fig. 2. The simulated return loss of the SIW cavity-backed slot antennas with : : , , different slot WLRs. ( , , ; : , , , , , , , ; , , , , : , , ; , , : , , , , ; and , , , all in mm.) ,
Fig. 5. The simulated return loss of the antenna with different lengths of the slotted-cavity.
Fig. 6. The simulated return loss of the antenna with different widths of the slotted-cavity.
E (y 0 z)
H (x 0 z)
Fig. 3. The simulated radiation patterns of the proposed antennas at 60 GHz and (b) -planes. with different WLRs in (a)
Fig. 7. The simulated return loss of the antenna with different slot lengths.
III. ANTENNA CHARACTERISTICS AND PARAMETRIC STUDY Fig. 4. The simulated gain of the proposed antennas with different slot WLRs.
WLR = 0 71
bandwidth is obtained. For the : , the top side of the slotted-cavity is nearly open with the wide slot, and the 10-dB return loss bandwidth reaches up to 11.6% (57–64 GHz). Fig. 3 presents the simulated radiation patterns of the cavity-backed slot antennas at 60 GHz with the WLRs of 0.2, 0.4, 0.6 and 0.71, respectively. It can be seen that the antennas keep consistent radiation patterns as varying the WLRs, and the higher gain is obtained for the bigger WLR under the same ground plane size of 10 mm 2 6 mm, as shown in Fig. 4.
The proposed cavity-backed slot antenna features the enhanced operating bandwidth by increasing the slot WLR where two resonances are well excited simultaneously when the slot WLR varies from 0.12 to 0.71 with consistent radiation performances. One of the resonances is generated by the radiating slot, where the SIW cavity functions as a reflector. The other one resonance is the resonant dominant mode of the slotted-cavity, where the slotted-cavity acts as a radiation element. Therefore, the slotted-cavity acts as not only a reflector but also a radiating element. In order to verify the mechanism of the dual-resonance behavior, a parametric study is carried out using the commercial software package, CST. The antenna with the WLR of 0.4 is discussed, and its parameters,
IEEE TRANSACTIONS ON ANTENNAS AND PROPAGATION, VOL. 60, NO. 12, DECEMBER 2012
WLR = 0 5
6025
WLR = 0 71
Fig. 10. The photograph of the fabricated 2 : and (c) : . (b)
2 4 arrays. (a) WLR = 0 12, :
Fig. 8. The simulated radiation patterns of the cavity-backed wide slot antenna with slot WLR of 0.71 at two resonant frequencies.
X = Y = 0:45 X = 0:5 = 1:8 L = 8 L = 2:15 L = 3:4
Fig. 11. (a) The simulated return loss and (b) the measured return loss of the , , arrays with the waveguide-to-SIW transition. ( , , , , , , , , , all in mm.).
Y = 0:4 d = 0:53 S = S = S W = 2:8 L = 1:9 W = 1:5
C. Length of the Transverse Slot Fig. 7 shows the variation of the return loss for different slot length
Ls while the WLR is fixed at 0.4. The lower resonance frequency shifts
down but the upper one maintains almost unchanged as the slot length is increased from 1.42 mm to 1.48 mm. These results indicate that the lower resonant frequency is mainly controlled by the slot length. On the other hand, the bandwidth is increased with the increased slot length.
Fig. 9. The geometry of the 2
2 4 antenna array prototypes.
unless otherwise stated, are fixed as Ls = 1:44 mm, d0 = 0:665 mm, d1 = 1:25 mm, Ww = 0:81 mm, and Wsiw1 = 2:2 mm. A. Length of the Slotted-Cavity Fig. 5 shows the effect of the length of the slotted-cavity, Wsiw1 on the impedance matching of the antenna. As Wsiw1 is varied from 1.92 mm to 2.07 mm, the upper resonant frequency shifts down and the lower one hardly changed leading to a narrower bandwidth. These results suggest that the upper resonance frequency can be controlled by adjusting the length of the slotted-cavity. B. Width of the Slotted-Cavity Fig. 6 shows the simulated return loss against the width of the slottedcavity. As the width, d1 , is varied from 1.24 mm to 1.27 mm, the upper resonance shifts down while the lower resonance is hardly changed. Moreover, it can be found that the 10-dB return loss impedance bandwidth of the antenna decreases from 6.5% for d1 = 1:24 mm to 5% for d1 = 1:27 mm with increasing d1 .
D. Discussion The parametric study reveals that the lower resonance results from the slot resonance by the wave transmission through the inductive window, and the upper resonance is a resonant mode of the slotted-cavity which is controlled by d1 and Wsiw1 . The radiation performances of the radiating slot and that of the slotted-cavity in the proposed antenna are expected to be consistent against the varying WLR of the slot. Fig. 8 shows the simulated radiation patterns of the cavity-backed wide slot antenna with the slot WLR of 0.71 at its two resonant frequencies. It is observed that the radiation patterns are very similar at the two different resonances. This attributes to the fact that the etched area on the broad-wall of the slotted-cavity can be modeled as a magnetic wall with the high-permittivity substrate [10], and similar field distributions in the slot and the magnetic wall will be excited by the feeding SIW with a TE10 mode. The simulated gain at the lower and upper resonance is 7.4 dBi @ 59.2 GHz and 7.9 dBi @ 62.8 GHz, respectively. As a result, the impedance and radiation bandwidths of the cavity-backed slot antenna can be enhanced by increasing the slot WLR. IV. ARRAY EXAMPLES WITH DIFFERENT WLRS OF SLOT Owning to the substrate in SIW, the proposed antennas feature compact size suitable for array application. In this section, three SIW
6026
IEEE TRANSACTIONS ON ANTENNAS AND PROPAGATION, VOL. 60, NO. 12, DECEMBER 2012
Fig. 12 shows measured gain of the antenna arrays. There is about 1-dB drop in the measured gains compared with the simulated ones, which may be caused by the dielectric loss of the substrate. The fluctuation of the measured gain of the three arrays is less than 2 dB over their operating bandwidth. The three arrays exhibit similar radiation patterns. Fig. 13 shows the measured normalized radiation patterns of the array with the WLR = 0:5. The 3-dB beamwidths in the E (y 0 z)- and H (x 0 z)-planes are 48000e and 18000e , respectively. Furthermore, the cross-polarization levels of less than 025 dB are observed in both the E - and H -planes. Fig. 12. (a) The simulated gain and (b) the measured gain of the arrays with different WLRs.
V. CONCLUSIONS Planar bandwidth enhanced wide slot antennas backed by an SIW cavity have been proposed and investigated at 60-GHz bands, where the backed cavity has played the role of not only a reflector but also a radiating element. By increasing the WLR of the transverse slot from 0.12 to 0.71, the impedance bandwidth has been enhanced from 3% to 11.6% with the introduction of dual-resonance operation. Over the enhanced bandwidth, the consistent radiation patterns have been achieved. The parametric study has been carried out to verify the dual-resonance behavior of the antenna. For comparison, three cavity-backed slot antenna arrays with the different WLRs have been designed and experimentally validated at 60-GHz bands using a standard PCB process.
Fig. 13. The simulated and measured normalized radiation patterns of the 2 4 array with the WLR of 0.5. (a) E (y z )-plane and (b) H (x z )-plane.
0
0
2
cavity-backed slot antenna arrays with the different WLRs are designed and prototyped on the RO3006 substrate using PCB technology at 60 GHz bands. Fig. 9 shows the geometry of these 2 2 4 antenna arrays, in which the feeding SIW is modified with the inductive via-hole. A compact eight-way tree-shape power divider splits the power into eight ways equally and excites all the elements in-phase. The antenna arrays are excited by a WR-15 waveguide through a stepped waveguide-to-SIW transition [11]. Fig. 10 shows the photographs of the prototypes with the WLRs of 0.12, 0.5 and 0.71, respectively. For the array with the WLR of 0.12, the distances between the adjacent elements are Lx = 3:3 mm (0:6600150 ) and Ly = 3:15 mm (0:6300150 ) along the x and y axis, respectively, where 00150 is the wavelength at 60 GHz in free space. For the arrays of WLR = 0:5 and 0.71, the distances are Lx = 4 mm (0:800150 ), Ly = 3:15 mm (0:6300150) and Lx = 3:45 mm (0:6900150), Ly = 3:37 mm (0:67400150), respectively. Fig. 11 shows the simulated and measured return loss of the antenna arrays with the waveguide-to-SIW transition. The measured return losses follow the trend of the simulated ones well with a slight frequency shifting which may be caused by the fabrication tolerance and the permittivity fluctuation. For the arrays of WLR = 0:12 and 0.5, the measured impedance bandwidths are 3.3% and 6.5%, respectively, which are consistent with the simulated results. For the array with WLR = 0:71, the simulated impedance bandwidth reaches up to 11.5%, whereas the measured return loss exhibits a slight deterioration, namely 9 dB at 57.3 GHz and 8.2 dB at 59.2 GHz, respectively. The array with the larger WLR is more sensitive to the fabrication tolerance, because the slot with a bigger WLR is closer to the inductive window and the via-holes. In practical applications, the antenna can be directly integrated into circuits, and it can be expected that the impedance matching and reliability of the antenna without any transition would be improved.
ACKNOWLEDGMENT The authors would like to thank J. Xu, Y. Zhang, W. Liu, T. S. Ping See, and Z. Kuai for their valuable discussions and kind help in fabrication and measurement.
REFERENCES [1] X. Yu, D. Ni, S. Liu, Z. Li, and W. Wang, “Design of a wideband waveguide slot array antenna and its decoupling method for synthetic aperture radar,” in Proc. Eur. Microwave Conf., 2008, pp. 135–138. [2] W. Hong, N. Behdad, and K. Sarabandi, “Size reduction of cavitybacked slot antennas,” IEEE Trans. Antennas Propag., vol. 54, no. 5, pp. 1461–1466, May 2006. [3] J. Hirokawa, H. Arai, and N. Goto, “Cavity-backed wide slot antenna,” IEE Proc., vol. 136, no. 1, pp. 29–33, Feb. 1989. [4] Y. Miura, J. Hirokawa, M. Ando, Y. Shibuya, and G. Yoshida, “Double-layer full-corporate-feed hollow-waveguide slot array antenna in the 60 GHz-band,” IEEE Trans. Antennas Propag., vol. 59, no. 8, pp. 2488–2851, Aug. 2011. [5] G. Q. Luo, Z. F. Hu, L. X. Dong, and L. L. Sun, “Planar slot antenna backed by substrate integrated waveguide cavity,” IEEE Antennas Wireless Propag. Lett., vol. 7, pp. 236–239, 2008. [6] J. C. Bohorquez, H. A. F. Pedraza, I. C. H. Pinzon, J. A. Castiblanco, N. Pena, and H. F. Guarnizo, “Planar substrate integrated waveguide cavity-backed antenna,” IEEE Antennas Wireless Propag. Lett., vol. 8, pp. 1139–1142, 2009. [7] G. Q. Luo, Z. F. Hu, Y. Liang, L. Y. Yu, and L. L. Sun, “Development of low profile cavity backed crossed slot antennas for planar integration,” IEEE Trans. Antennas Propag., vol. 57, no. 10, pp. 2972–2979, Oct. 2009. [8] X. Chen, K. Wu, L. Han, and F. He, “Low-cost high gain planar antenna array for 60-GHz band applications,” IEEE Trans. Antennas Propag., vol. 58, no. 6, pp. 2126–2129, June 2010. [9] F. Xu and K. Wu, “Guided-wave and leakage characteristics of substrate integrated waveguide,” IEEE Trans. Microw. Theory Tech., vol. 53, no. 1, pp. 66–73, Jan. 2005. [10] A. S. Al-Zoubi, A. A. Kishk, and A. W. Glisson, “A linear rectangular dielectric resonator antenna array fed by dielectric image guide with low cross polarization,” IEEE Trans. Antennas Propag., vol. 58, no. 3, pp. 697–705, Mar. 2010. [11] T. Kai, J. Hirokawa, and M. Ando, “A stepped post-wall waveguide with aperture interface to standard waveguide,” in Proc. Antennas and Propagation Soc. Int. Symp., 2004, vol. 2, pp. 1527–1530.
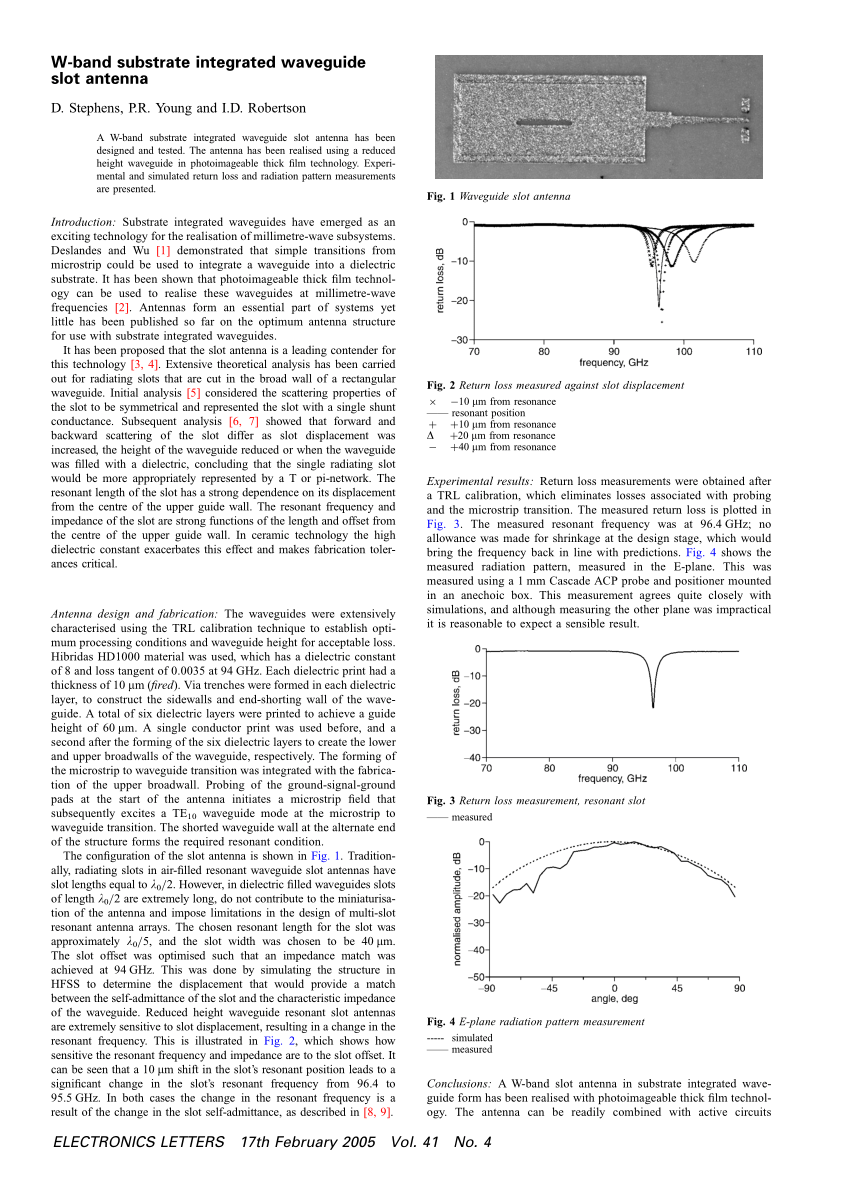
Topology of the substrate integrated waveguide. In this paper, we will propose, design of waveguide slots array antennas based on the SIW technology, the array consists of two longitudinal slots and it is match terminated. Firstly, we give the design equations for tapered microstrip-SIW transitions. A novel shunt longitudinal slot planar array antenna based on a ridged substrate integrated waveguide (RSIW) with a good cross polarization and isolation is designed and fabricated. The slots in.

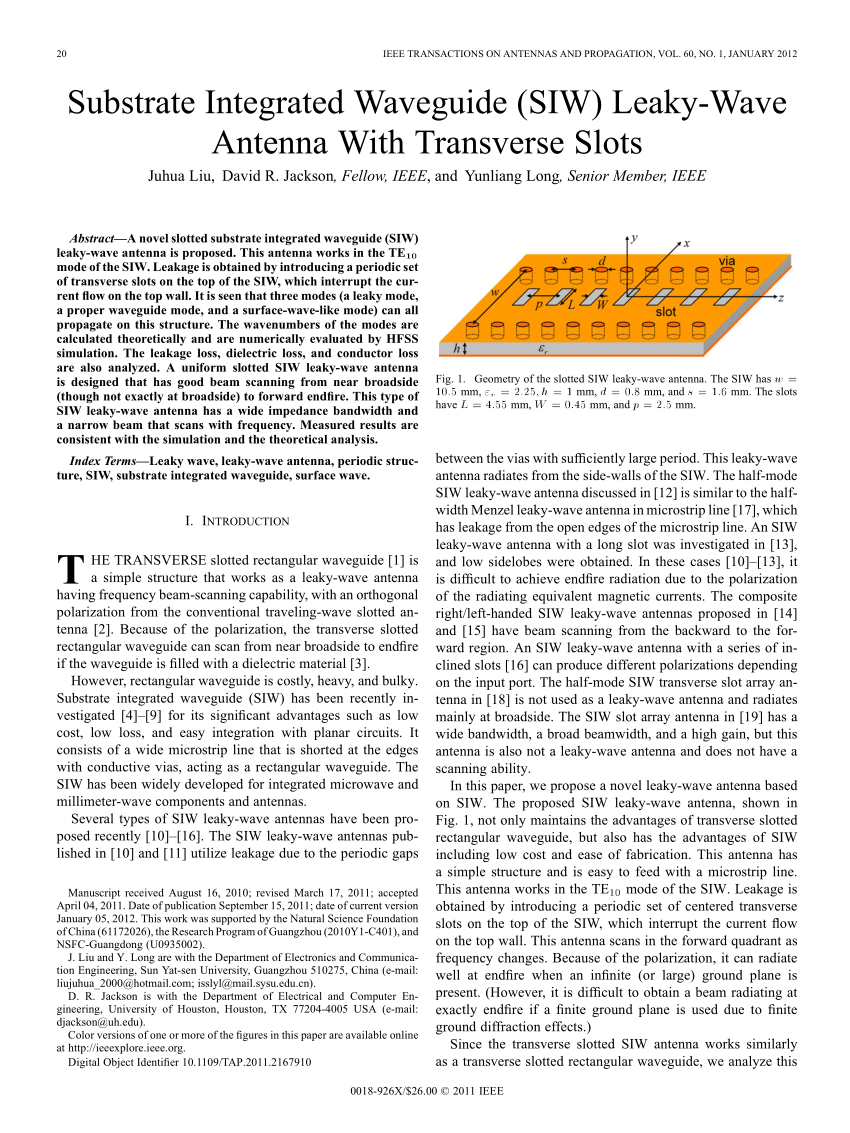
Substrate Integrated Waveguide Filters
IEEE TRANSACTIONS ON ANTENNAS AND PROPAGATION, VOL. 60, NO. 12, DECEMBER 2012
6023
[11] R. Liu, A. Degrion, J. J. Mock, and D. R. Smith, “Negative index material composed of electric and magnetic resonators,” Appl. Phys. Lett., vol. 90, no. 26, Jun. 2007. [12] Z. Szabó, G.-H. Park, R. Hedge, and E.-P. Li, “A unique extraction of metamaterial parameters based on Kramers-Kronig relationship,” IEEE Trans. Microwave Theory Tech., vol. 58, no. 10, pp. 2646–2653, Oct. 2010. [13] V. Lucarini, J. J. Saarinen, K. E. Peiponen, and E. M. Vartiainen, Kramers-Kronig Relations in Optical Materials Research. Berlin, Germany: Springer-Verlag, 2005.
Substrate Integrated Waveguide Cavity-Backed Wide Slot Antenna for 60-GHz Bands Ke Gong, Zhi Ning Chen, Xianming Qing, Peng Chen, and Wei Hong
Abstract—A wide slot antenna backed by a substrate integrated waveguide (SIW) cavity is presented and investigated for the enhancement of operating bandwidth at 60-GHz band. The 10-dB return loss impedance bandwidth of the antenna increases from 3% to 11.6% with consistent radiation performance as the width to length ratio (WLR) of the rectangular slot cutting from the SIW increases from 0.12 to 0.71. Its dual-resonance 4 anoperation mechanism is verified by a parametric study. Three 2 tenna arrays with different slot WLRs are designed and fabricated with a printed circuit board (PCB) process at the 60-GHz bands. The measured results show that the antenna array with slot WLR of 0.71 achieves the gain of 10–12 dBi and the cross-polarization levels of better than 25 dB in both - and -planes over the enhanced operating bandwidth of about 11.6%. Index Terms—Cavity-backed antenna, dual-resonance, slot antenna, substrate integrated waveguide (SIW), 60-GHz band.
I. INTRODUCTION Waveguide slot array antennas and cavity-backed slot antennas are widely used in radar and communication systems. The slot with a large width-to-length ratio has been successfully employed to enhance the operating bandwidth of the antennas [1]–[4]. On the other hand, substrate integrated waveguide (SIW) slot array antennas and cavity-backed slot antennas have been extremely investigated in recent years. However, the reduced waveguide height of the SIW greatly Manuscript received March 31, 2012; revised June 06, 2012; accepted July 30, 2012. Date of publication August 14, 2012; date of current version November 29, 2012. This work was supported in part by the National 973 project of China 2010CB327400, the National Nature Science Foundation of China (NSFC) under Grant 60921063, Research Fund for the Doctoral Program of Higher Education of China under Grant 20100092120013 and in part by the Agency for Science, Technology and Research (A*STAR), Singapore, Terahertz Science & Technology Inter-RI Program under Grant #0821410040 by the Institute for Infocomm Research, Agency for Science, Technology and Research (A*STAR), Singapore. K. Gong, P. Chen and W. Hong are with the State Key Laboratory of Millimeter Waves, Southeast University, Nanjing 210096, China (e-mail: [email protected]; [email protected]; [email protected]). Z. N. Chen is with Department of Electrical and Computer Engineering, National University of Singapore, Singapore 117583 and also with the Institute for Infocomm Research, Agency for Science, Technology and Research (A*STAR), 138632, Singapore (e-mail: [email protected]; [email protected]). X. Qing is with the Institute for Infocomm Research, Agency for Science, Technology and Research (A*STAR), 138632, Singapore (e-mail: [email protected]). Color versions of one or more of the figures in this communication are available online at http://ieeexplore.ieee.org. Digital Object Identifier 10.1109/TAP.2012.2213060
Fig. 1. The SIW cavity-backed wide slot antenna with slot
WLR = 0 4. :
narrows the impedance bandwidth of the antennas to around 3% or less [5]–[8]. In this communication, a planar wide slot antenna backed by an SIW cavity is proposed for bandwidth enhancement at 60-GHz bands. The impedance bandwidth, radiation patterns and gain of the antenna are analyzed against the WLR of the slot. In the proposed antenna, the backed cavity is not only a reflector for the radiating slot, but also a radiation element for the whole antenna. To verify the dual-resonance operating mechanism of the antenna, a parametric study is carried out using CST. The cavity-backed slot antennas show compact size, which are suitable for array application. With the optimized elements, three 2 2 4 antenna arrays with different WLRs are prototyped using PCB technology at 60 GHz bands. II. SIW CAVITY-BACKED WIDE SLOT ANTENNA Fig. 1 depicts the geometry of the proposed SIW cavity-backed wide slot antenna. A transverse wide slot with the WLR of 0.4 ( s s = 0 4) is etched onto the broad-wall of an SIW. The SIW is short-circuited at a distance 0 from the center of the slot. An inductive window is inserted into the SIW at a distance 1 from the short-circuited end of SIW, where 1 is about twice of 0 . Thus, an SIW cavity-backed wide slot antenna is formed with size of siw1 and 1 , and it is fed by the SIW with the width of siw . 1 and are the diameter of the via-hole and the spacing between adjacent via-holes, respectively. The slotted-cavity and the feeding SIW are formed with the conditions of 0 5 and 1 0 0 1 [9]. 1 In the proposed antenna, the slot operates at its dominant mode with the overall length of approximate a half operating wavelength, and the backed slotted-cavity resonates at its dominant mode frequency. The antenna is fabricated onto a piece of a 0.635-mm thick RO3006 substrate with a dielectric constant of r = 6 15 and loss tangent of tan = 0 0025, and simulated and optimized using CST. The proposed cavity-backed slot antenna exhibits a dual-resonant characteristic, and its operating bandwidth can be enhanced by increasing the slot WLR. Fig. 2 shows the simulated return losses of the antennas with the WLRs of 0.2, 0.4, 0.6 and 0.71, respectively. During the WLR varies from 0.2 to 0.71, the good impedance matching with two resonances is maintained by tuning the parameters including w , and nearly four times enhancement in s, 1, siw1 , 0 and
:
d
:
D =P 000e
W =L
d
d d W W D P
D =0015
:
'
:
L d W
d
d
W
:
6024
IEEE TRANSACTIONS ON ANTENNAS AND PROPAGATION, VOL. 60, NO. 12, DECEMBER 2012
WLR = 0 2 W = W = 1:85 L = 1:33 W = 0:925 d = 0:665 d = 1:25 d = 1:375 WLR = 0:4 W = 1:85 W = 2 L = 1:43 W = 0:99 d = 0:69 d = 1:3 d = 1:35 WLR = 0:6 W = 1:85 W = 2:05 L = 1:55 W = 1 d = 0:755 d = 1:45 d = 1:25 WLR = 0:71 W = 1:85 W = 1:95 L = 1:65 W = 1:13 d = 0:735 d = 1:47 d = 1:24 D = 0:2 P = 0:4 W = 6 L = 10
Fig. 2. The simulated return loss of the SIW cavity-backed slot antennas with : : , , different slot WLRs. ( , , ; : , , , , , , , ; , , , , : , , ; , , : , , , , ; and , , , all in mm.) ,
Fig. 5. The simulated return loss of the antenna with different lengths of the slotted-cavity.
Fig. 6. The simulated return loss of the antenna with different widths of the slotted-cavity.
E (y 0 z)
H (x 0 z)
Fig. 3. The simulated radiation patterns of the proposed antennas at 60 GHz and (b) -planes. with different WLRs in (a)
Fig. 7. The simulated return loss of the antenna with different slot lengths.
III. ANTENNA CHARACTERISTICS AND PARAMETRIC STUDY Fig. 4. The simulated gain of the proposed antennas with different slot WLRs.
WLR = 0 71
bandwidth is obtained. For the : , the top side of the slotted-cavity is nearly open with the wide slot, and the 10-dB return loss bandwidth reaches up to 11.6% (57–64 GHz). Fig. 3 presents the simulated radiation patterns of the cavity-backed slot antennas at 60 GHz with the WLRs of 0.2, 0.4, 0.6 and 0.71, respectively. It can be seen that the antennas keep consistent radiation patterns as varying the WLRs, and the higher gain is obtained for the bigger WLR under the same ground plane size of 10 mm 2 6 mm, as shown in Fig. 4.
The proposed cavity-backed slot antenna features the enhanced operating bandwidth by increasing the slot WLR where two resonances are well excited simultaneously when the slot WLR varies from 0.12 to 0.71 with consistent radiation performances. One of the resonances is generated by the radiating slot, where the SIW cavity functions as a reflector. The other one resonance is the resonant dominant mode of the slotted-cavity, where the slotted-cavity acts as a radiation element. Therefore, the slotted-cavity acts as not only a reflector but also a radiating element. In order to verify the mechanism of the dual-resonance behavior, a parametric study is carried out using the commercial software package, CST. The antenna with the WLR of 0.4 is discussed, and its parameters,
IEEE TRANSACTIONS ON ANTENNAS AND PROPAGATION, VOL. 60, NO. 12, DECEMBER 2012
WLR = 0 5
6025
WLR = 0 71
Fig. 10. The photograph of the fabricated 2 : and (c) : . (b)
2 4 arrays. (a) WLR = 0 12, :
Fig. 8. The simulated radiation patterns of the cavity-backed wide slot antenna with slot WLR of 0.71 at two resonant frequencies.
X = Y = 0:45 X = 0:5 = 1:8 L = 8 L = 2:15 L = 3:4
Fig. 11. (a) The simulated return loss and (b) the measured return loss of the , , arrays with the waveguide-to-SIW transition. ( , , , , , , , , , all in mm.).
Y = 0:4 d = 0:53 S = S = S W = 2:8 L = 1:9 W = 1:5
C. Length of the Transverse Slot Fig. 7 shows the variation of the return loss for different slot length
Ls while the WLR is fixed at 0.4. The lower resonance frequency shifts
down but the upper one maintains almost unchanged as the slot length is increased from 1.42 mm to 1.48 mm. These results indicate that the lower resonant frequency is mainly controlled by the slot length. On the other hand, the bandwidth is increased with the increased slot length.
Fig. 9. The geometry of the 2
2 4 antenna array prototypes.
unless otherwise stated, are fixed as Ls = 1:44 mm, d0 = 0:665 mm, d1 = 1:25 mm, Ww = 0:81 mm, and Wsiw1 = 2:2 mm. A. Length of the Slotted-Cavity Fig. 5 shows the effect of the length of the slotted-cavity, Wsiw1 on the impedance matching of the antenna. As Wsiw1 is varied from 1.92 mm to 2.07 mm, the upper resonant frequency shifts down and the lower one hardly changed leading to a narrower bandwidth. These results suggest that the upper resonance frequency can be controlled by adjusting the length of the slotted-cavity. B. Width of the Slotted-Cavity Fig. 6 shows the simulated return loss against the width of the slottedcavity. As the width, d1 , is varied from 1.24 mm to 1.27 mm, the upper resonance shifts down while the lower resonance is hardly changed. Moreover, it can be found that the 10-dB return loss impedance bandwidth of the antenna decreases from 6.5% for d1 = 1:24 mm to 5% for d1 = 1:27 mm with increasing d1 .
D. Discussion The parametric study reveals that the lower resonance results from the slot resonance by the wave transmission through the inductive window, and the upper resonance is a resonant mode of the slotted-cavity which is controlled by d1 and Wsiw1 . The radiation performances of the radiating slot and that of the slotted-cavity in the proposed antenna are expected to be consistent against the varying WLR of the slot. Fig. 8 shows the simulated radiation patterns of the cavity-backed wide slot antenna with the slot WLR of 0.71 at its two resonant frequencies. It is observed that the radiation patterns are very similar at the two different resonances. This attributes to the fact that the etched area on the broad-wall of the slotted-cavity can be modeled as a magnetic wall with the high-permittivity substrate [10], and similar field distributions in the slot and the magnetic wall will be excited by the feeding SIW with a TE10 mode. The simulated gain at the lower and upper resonance is 7.4 dBi @ 59.2 GHz and 7.9 dBi @ 62.8 GHz, respectively. As a result, the impedance and radiation bandwidths of the cavity-backed slot antenna can be enhanced by increasing the slot WLR. IV. ARRAY EXAMPLES WITH DIFFERENT WLRS OF SLOT Owning to the substrate in SIW, the proposed antennas feature compact size suitable for array application. In this section, three SIW
6026
IEEE TRANSACTIONS ON ANTENNAS AND PROPAGATION, VOL. 60, NO. 12, DECEMBER 2012
Fig. 12 shows measured gain of the antenna arrays. There is about 1-dB drop in the measured gains compared with the simulated ones, which may be caused by the dielectric loss of the substrate. The fluctuation of the measured gain of the three arrays is less than 2 dB over their operating bandwidth. The three arrays exhibit similar radiation patterns. Fig. 13 shows the measured normalized radiation patterns of the array with the WLR = 0:5. The 3-dB beamwidths in the E (y 0 z)- and H (x 0 z)-planes are 48000e and 18000e , respectively. Furthermore, the cross-polarization levels of less than 025 dB are observed in both the E - and H -planes. Fig. 12. (a) The simulated gain and (b) the measured gain of the arrays with different WLRs.
V. CONCLUSIONS Planar bandwidth enhanced wide slot antennas backed by an SIW cavity have been proposed and investigated at 60-GHz bands, where the backed cavity has played the role of not only a reflector but also a radiating element. By increasing the WLR of the transverse slot from 0.12 to 0.71, the impedance bandwidth has been enhanced from 3% to 11.6% with the introduction of dual-resonance operation. Over the enhanced bandwidth, the consistent radiation patterns have been achieved. The parametric study has been carried out to verify the dual-resonance behavior of the antenna. For comparison, three cavity-backed slot antenna arrays with the different WLRs have been designed and experimentally validated at 60-GHz bands using a standard PCB process.
Fig. 13. The simulated and measured normalized radiation patterns of the 2 4 array with the WLR of 0.5. (a) E (y z )-plane and (b) H (x z )-plane.
0
0
2
cavity-backed slot antenna arrays with the different WLRs are designed and prototyped on the RO3006 substrate using PCB technology at 60 GHz bands. Fig. 9 shows the geometry of these 2 2 4 antenna arrays, in which the feeding SIW is modified with the inductive via-hole. A compact eight-way tree-shape power divider splits the power into eight ways equally and excites all the elements in-phase. The antenna arrays are excited by a WR-15 waveguide through a stepped waveguide-to-SIW transition [11]. Fig. 10 shows the photographs of the prototypes with the WLRs of 0.12, 0.5 and 0.71, respectively. For the array with the WLR of 0.12, the distances between the adjacent elements are Lx = 3:3 mm (0:6600150 ) and Ly = 3:15 mm (0:6300150 ) along the x and y axis, respectively, where 00150 is the wavelength at 60 GHz in free space. For the arrays of WLR = 0:5 and 0.71, the distances are Lx = 4 mm (0:800150 ), Ly = 3:15 mm (0:6300150) and Lx = 3:45 mm (0:6900150), Ly = 3:37 mm (0:67400150), respectively. Fig. 11 shows the simulated and measured return loss of the antenna arrays with the waveguide-to-SIW transition. The measured return losses follow the trend of the simulated ones well with a slight frequency shifting which may be caused by the fabrication tolerance and the permittivity fluctuation. For the arrays of WLR = 0:12 and 0.5, the measured impedance bandwidths are 3.3% and 6.5%, respectively, which are consistent with the simulated results. For the array with WLR = 0:71, the simulated impedance bandwidth reaches up to 11.5%, whereas the measured return loss exhibits a slight deterioration, namely 9 dB at 57.3 GHz and 8.2 dB at 59.2 GHz, respectively. The array with the larger WLR is more sensitive to the fabrication tolerance, because the slot with a bigger WLR is closer to the inductive window and the via-holes. In practical applications, the antenna can be directly integrated into circuits, and it can be expected that the impedance matching and reliability of the antenna without any transition would be improved.
ACKNOWLEDGMENT The authors would like to thank J. Xu, Y. Zhang, W. Liu, T. S. Ping See, and Z. Kuai for their valuable discussions and kind help in fabrication and measurement.
REFERENCES [1] X. Yu, D. Ni, S. Liu, Z. Li, and W. Wang, “Design of a wideband waveguide slot array antenna and its decoupling method for synthetic aperture radar,” in Proc. Eur. Microwave Conf., 2008, pp. 135–138. [2] W. Hong, N. Behdad, and K. Sarabandi, “Size reduction of cavitybacked slot antennas,” IEEE Trans. Antennas Propag., vol. 54, no. 5, pp. 1461–1466, May 2006. [3] J. Hirokawa, H. Arai, and N. Goto, “Cavity-backed wide slot antenna,” IEE Proc., vol. 136, no. 1, pp. 29–33, Feb. 1989. [4] Y. Miura, J. Hirokawa, M. Ando, Y. Shibuya, and G. Yoshida, “Double-layer full-corporate-feed hollow-waveguide slot array antenna in the 60 GHz-band,” IEEE Trans. Antennas Propag., vol. 59, no. 8, pp. 2488–2851, Aug. 2011. [5] G. Q. Luo, Z. F. Hu, L. X. Dong, and L. L. Sun, “Planar slot antenna backed by substrate integrated waveguide cavity,” IEEE Antennas Wireless Propag. Lett., vol. 7, pp. 236–239, 2008. [6] J. C. Bohorquez, H. A. F. Pedraza, I. C. H. Pinzon, J. A. Castiblanco, N. Pena, and H. F. Guarnizo, “Planar substrate integrated waveguide cavity-backed antenna,” IEEE Antennas Wireless Propag. Lett., vol. 8, pp. 1139–1142, 2009. [7] G. Q. Luo, Z. F. Hu, Y. Liang, L. Y. Yu, and L. L. Sun, “Development of low profile cavity backed crossed slot antennas for planar integration,” IEEE Trans. Antennas Propag., vol. 57, no. 10, pp. 2972–2979, Oct. 2009. [8] X. Chen, K. Wu, L. Han, and F. He, “Low-cost high gain planar antenna array for 60-GHz band applications,” IEEE Trans. Antennas Propag., vol. 58, no. 6, pp. 2126–2129, June 2010. [9] F. Xu and K. Wu, “Guided-wave and leakage characteristics of substrate integrated waveguide,” IEEE Trans. Microw. Theory Tech., vol. 53, no. 1, pp. 66–73, Jan. 2005. [10] A. S. Al-Zoubi, A. A. Kishk, and A. W. Glisson, “A linear rectangular dielectric resonator antenna array fed by dielectric image guide with low cross polarization,” IEEE Trans. Antennas Propag., vol. 58, no. 3, pp. 697–705, Mar. 2010. [11] T. Kai, J. Hirokawa, and M. Ando, “A stepped post-wall waveguide with aperture interface to standard waveguide,” in Proc. Antennas and Propagation Soc. Int. Symp., 2004, vol. 2, pp. 1527–1530.
6023
[11] R. Liu, A. Degrion, J. J. Mock, and D. R. Smith, “Negative index material composed of electric and magnetic resonators,” Appl. Phys. Lett., vol. 90, no. 26, Jun. 2007. [12] Z. Szabó, G.-H. Park, R. Hedge, and E.-P. Li, “A unique extraction of metamaterial parameters based on Kramers-Kronig relationship,” IEEE Trans. Microwave Theory Tech., vol. 58, no. 10, pp. 2646–2653, Oct. 2010. [13] V. Lucarini, J. J. Saarinen, K. E. Peiponen, and E. M. Vartiainen, Kramers-Kronig Relations in Optical Materials Research. Berlin, Germany: Springer-Verlag, 2005.
Substrate Integrated Waveguide Cavity-Backed Wide Slot Antenna for 60-GHz Bands Ke Gong, Zhi Ning Chen, Xianming Qing, Peng Chen, and Wei Hong
Abstract—A wide slot antenna backed by a substrate integrated waveguide (SIW) cavity is presented and investigated for the enhancement of operating bandwidth at 60-GHz band. The 10-dB return loss impedance bandwidth of the antenna increases from 3% to 11.6% with consistent radiation performance as the width to length ratio (WLR) of the rectangular slot cutting from the SIW increases from 0.12 to 0.71. Its dual-resonance 4 anoperation mechanism is verified by a parametric study. Three 2 tenna arrays with different slot WLRs are designed and fabricated with a printed circuit board (PCB) process at the 60-GHz bands. The measured results show that the antenna array with slot WLR of 0.71 achieves the gain of 10–12 dBi and the cross-polarization levels of better than 25 dB in both - and -planes over the enhanced operating bandwidth of about 11.6%. Index Terms—Cavity-backed antenna, dual-resonance, slot antenna, substrate integrated waveguide (SIW), 60-GHz band.
I. INTRODUCTION Waveguide slot array antennas and cavity-backed slot antennas are widely used in radar and communication systems. The slot with a large width-to-length ratio has been successfully employed to enhance the operating bandwidth of the antennas [1]–[4]. On the other hand, substrate integrated waveguide (SIW) slot array antennas and cavity-backed slot antennas have been extremely investigated in recent years. However, the reduced waveguide height of the SIW greatly Manuscript received March 31, 2012; revised June 06, 2012; accepted July 30, 2012. Date of publication August 14, 2012; date of current version November 29, 2012. This work was supported in part by the National 973 project of China 2010CB327400, the National Nature Science Foundation of China (NSFC) under Grant 60921063, Research Fund for the Doctoral Program of Higher Education of China under Grant 20100092120013 and in part by the Agency for Science, Technology and Research (A*STAR), Singapore, Terahertz Science & Technology Inter-RI Program under Grant #0821410040 by the Institute for Infocomm Research, Agency for Science, Technology and Research (A*STAR), Singapore. K. Gong, P. Chen and W. Hong are with the State Key Laboratory of Millimeter Waves, Southeast University, Nanjing 210096, China (e-mail: [email protected]; [email protected]; [email protected]). Z. N. Chen is with Department of Electrical and Computer Engineering, National University of Singapore, Singapore 117583 and also with the Institute for Infocomm Research, Agency for Science, Technology and Research (A*STAR), 138632, Singapore (e-mail: [email protected]; [email protected]). X. Qing is with the Institute for Infocomm Research, Agency for Science, Technology and Research (A*STAR), 138632, Singapore (e-mail: [email protected]). Color versions of one or more of the figures in this communication are available online at http://ieeexplore.ieee.org. Digital Object Identifier 10.1109/TAP.2012.2213060
Fig. 1. The SIW cavity-backed wide slot antenna with slot
WLR = 0 4. :
narrows the impedance bandwidth of the antennas to around 3% or less [5]–[8]. In this communication, a planar wide slot antenna backed by an SIW cavity is proposed for bandwidth enhancement at 60-GHz bands. The impedance bandwidth, radiation patterns and gain of the antenna are analyzed against the WLR of the slot. In the proposed antenna, the backed cavity is not only a reflector for the radiating slot, but also a radiation element for the whole antenna. To verify the dual-resonance operating mechanism of the antenna, a parametric study is carried out using CST. The cavity-backed slot antennas show compact size, which are suitable for array application. With the optimized elements, three 2 2 4 antenna arrays with different WLRs are prototyped using PCB technology at 60 GHz bands. II. SIW CAVITY-BACKED WIDE SLOT ANTENNA Fig. 1 depicts the geometry of the proposed SIW cavity-backed wide slot antenna. A transverse wide slot with the WLR of 0.4 ( s s = 0 4) is etched onto the broad-wall of an SIW. The SIW is short-circuited at a distance 0 from the center of the slot. An inductive window is inserted into the SIW at a distance 1 from the short-circuited end of SIW, where 1 is about twice of 0 . Thus, an SIW cavity-backed wide slot antenna is formed with size of siw1 and 1 , and it is fed by the SIW with the width of siw . 1 and are the diameter of the via-hole and the spacing between adjacent via-holes, respectively. The slotted-cavity and the feeding SIW are formed with the conditions of 0 5 and 1 0 0 1 [9]. 1 In the proposed antenna, the slot operates at its dominant mode with the overall length of approximate a half operating wavelength, and the backed slotted-cavity resonates at its dominant mode frequency. The antenna is fabricated onto a piece of a 0.635-mm thick RO3006 substrate with a dielectric constant of r = 6 15 and loss tangent of tan = 0 0025, and simulated and optimized using CST. The proposed cavity-backed slot antenna exhibits a dual-resonant characteristic, and its operating bandwidth can be enhanced by increasing the slot WLR. Fig. 2 shows the simulated return losses of the antennas with the WLRs of 0.2, 0.4, 0.6 and 0.71, respectively. During the WLR varies from 0.2 to 0.71, the good impedance matching with two resonances is maintained by tuning the parameters including w , and nearly four times enhancement in s, 1, siw1 , 0 and
:
d
:
D =P 000e
W =L
d
d d W W D P
D =0015
:
'
:
L d W
d
d
W
:
6024
IEEE TRANSACTIONS ON ANTENNAS AND PROPAGATION, VOL. 60, NO. 12, DECEMBER 2012
WLR = 0 2 W = W = 1:85 L = 1:33 W = 0:925 d = 0:665 d = 1:25 d = 1:375 WLR = 0:4 W = 1:85 W = 2 L = 1:43 W = 0:99 d = 0:69 d = 1:3 d = 1:35 WLR = 0:6 W = 1:85 W = 2:05 L = 1:55 W = 1 d = 0:755 d = 1:45 d = 1:25 WLR = 0:71 W = 1:85 W = 1:95 L = 1:65 W = 1:13 d = 0:735 d = 1:47 d = 1:24 D = 0:2 P = 0:4 W = 6 L = 10
Fig. 2. The simulated return loss of the SIW cavity-backed slot antennas with : : , , different slot WLRs. ( , , ; : , , , , , , , ; , , , , : , , ; , , : , , , , ; and , , , all in mm.) ,
Fig. 5. The simulated return loss of the antenna with different lengths of the slotted-cavity.
Fig. 6. The simulated return loss of the antenna with different widths of the slotted-cavity.
E (y 0 z)
H (x 0 z)
Fig. 3. The simulated radiation patterns of the proposed antennas at 60 GHz and (b) -planes. with different WLRs in (a)
Fig. 7. The simulated return loss of the antenna with different slot lengths.
III. ANTENNA CHARACTERISTICS AND PARAMETRIC STUDY Fig. 4. The simulated gain of the proposed antennas with different slot WLRs.
WLR = 0 71
bandwidth is obtained. For the : , the top side of the slotted-cavity is nearly open with the wide slot, and the 10-dB return loss bandwidth reaches up to 11.6% (57–64 GHz). Fig. 3 presents the simulated radiation patterns of the cavity-backed slot antennas at 60 GHz with the WLRs of 0.2, 0.4, 0.6 and 0.71, respectively. It can be seen that the antennas keep consistent radiation patterns as varying the WLRs, and the higher gain is obtained for the bigger WLR under the same ground plane size of 10 mm 2 6 mm, as shown in Fig. 4.
The proposed cavity-backed slot antenna features the enhanced operating bandwidth by increasing the slot WLR where two resonances are well excited simultaneously when the slot WLR varies from 0.12 to 0.71 with consistent radiation performances. One of the resonances is generated by the radiating slot, where the SIW cavity functions as a reflector. The other one resonance is the resonant dominant mode of the slotted-cavity, where the slotted-cavity acts as a radiation element. Therefore, the slotted-cavity acts as not only a reflector but also a radiating element. In order to verify the mechanism of the dual-resonance behavior, a parametric study is carried out using the commercial software package, CST. The antenna with the WLR of 0.4 is discussed, and its parameters,
IEEE TRANSACTIONS ON ANTENNAS AND PROPAGATION, VOL. 60, NO. 12, DECEMBER 2012
WLR = 0 5
6025
WLR = 0 71
Fig. 10. The photograph of the fabricated 2 : and (c) : . (b)
2 4 arrays. (a) WLR = 0 12, :
Fig. 8. The simulated radiation patterns of the cavity-backed wide slot antenna with slot WLR of 0.71 at two resonant frequencies.
X = Y = 0:45 X = 0:5 = 1:8 L = 8 L = 2:15 L = 3:4
Fig. 11. (a) The simulated return loss and (b) the measured return loss of the , , arrays with the waveguide-to-SIW transition. ( , , , , , , , , , all in mm.).
Y = 0:4 d = 0:53 S = S = S W = 2:8 L = 1:9 W = 1:5
C. Length of the Transverse Slot Fig. 7 shows the variation of the return loss for different slot length
Ls while the WLR is fixed at 0.4. The lower resonance frequency shifts
down but the upper one maintains almost unchanged as the slot length is increased from 1.42 mm to 1.48 mm. These results indicate that the lower resonant frequency is mainly controlled by the slot length. On the other hand, the bandwidth is increased with the increased slot length.
Fig. 9. The geometry of the 2
2 4 antenna array prototypes.
unless otherwise stated, are fixed as Ls = 1:44 mm, d0 = 0:665 mm, d1 = 1:25 mm, Ww = 0:81 mm, and Wsiw1 = 2:2 mm. A. Length of the Slotted-Cavity Fig. 5 shows the effect of the length of the slotted-cavity, Wsiw1 on the impedance matching of the antenna. As Wsiw1 is varied from 1.92 mm to 2.07 mm, the upper resonant frequency shifts down and the lower one hardly changed leading to a narrower bandwidth. These results suggest that the upper resonance frequency can be controlled by adjusting the length of the slotted-cavity. B. Width of the Slotted-Cavity Fig. 6 shows the simulated return loss against the width of the slottedcavity. As the width, d1 , is varied from 1.24 mm to 1.27 mm, the upper resonance shifts down while the lower resonance is hardly changed. Moreover, it can be found that the 10-dB return loss impedance bandwidth of the antenna decreases from 6.5% for d1 = 1:24 mm to 5% for d1 = 1:27 mm with increasing d1 .
D. Discussion The parametric study reveals that the lower resonance results from the slot resonance by the wave transmission through the inductive window, and the upper resonance is a resonant mode of the slotted-cavity which is controlled by d1 and Wsiw1 . The radiation performances of the radiating slot and that of the slotted-cavity in the proposed antenna are expected to be consistent against the varying WLR of the slot. Fig. 8 shows the simulated radiation patterns of the cavity-backed wide slot antenna with the slot WLR of 0.71 at its two resonant frequencies. It is observed that the radiation patterns are very similar at the two different resonances. This attributes to the fact that the etched area on the broad-wall of the slotted-cavity can be modeled as a magnetic wall with the high-permittivity substrate [10], and similar field distributions in the slot and the magnetic wall will be excited by the feeding SIW with a TE10 mode. The simulated gain at the lower and upper resonance is 7.4 dBi @ 59.2 GHz and 7.9 dBi @ 62.8 GHz, respectively. As a result, the impedance and radiation bandwidths of the cavity-backed slot antenna can be enhanced by increasing the slot WLR. IV. ARRAY EXAMPLES WITH DIFFERENT WLRS OF SLOT Owning to the substrate in SIW, the proposed antennas feature compact size suitable for array application. In this section, three SIW
6026
IEEE TRANSACTIONS ON ANTENNAS AND PROPAGATION, VOL. 60, NO. 12, DECEMBER 2012
Fig. 12 shows measured gain of the antenna arrays. There is about 1-dB drop in the measured gains compared with the simulated ones, which may be caused by the dielectric loss of the substrate. The fluctuation of the measured gain of the three arrays is less than 2 dB over their operating bandwidth. The three arrays exhibit similar radiation patterns. Fig. 13 shows the measured normalized radiation patterns of the array with the WLR = 0:5. The 3-dB beamwidths in the E (y 0 z)- and H (x 0 z)-planes are 48000e and 18000e , respectively. Furthermore, the cross-polarization levels of less than 025 dB are observed in both the E - and H -planes. Fig. 12. (a) The simulated gain and (b) the measured gain of the arrays with different WLRs.
V. CONCLUSIONS Planar bandwidth enhanced wide slot antennas backed by an SIW cavity have been proposed and investigated at 60-GHz bands, where the backed cavity has played the role of not only a reflector but also a radiating element. By increasing the WLR of the transverse slot from 0.12 to 0.71, the impedance bandwidth has been enhanced from 3% to 11.6% with the introduction of dual-resonance operation. Over the enhanced bandwidth, the consistent radiation patterns have been achieved. The parametric study has been carried out to verify the dual-resonance behavior of the antenna. For comparison, three cavity-backed slot antenna arrays with the different WLRs have been designed and experimentally validated at 60-GHz bands using a standard PCB process.
Fig. 13. The simulated and measured normalized radiation patterns of the 2 4 array with the WLR of 0.5. (a) E (y z )-plane and (b) H (x z )-plane.
0
0
2
cavity-backed slot antenna arrays with the different WLRs are designed and prototyped on the RO3006 substrate using PCB technology at 60 GHz bands. Fig. 9 shows the geometry of these 2 2 4 antenna arrays, in which the feeding SIW is modified with the inductive via-hole. A compact eight-way tree-shape power divider splits the power into eight ways equally and excites all the elements in-phase. The antenna arrays are excited by a WR-15 waveguide through a stepped waveguide-to-SIW transition [11]. Fig. 10 shows the photographs of the prototypes with the WLRs of 0.12, 0.5 and 0.71, respectively. For the array with the WLR of 0.12, the distances between the adjacent elements are Lx = 3:3 mm (0:6600150 ) and Ly = 3:15 mm (0:6300150 ) along the x and y axis, respectively, where 00150 is the wavelength at 60 GHz in free space. For the arrays of WLR = 0:5 and 0.71, the distances are Lx = 4 mm (0:800150 ), Ly = 3:15 mm (0:6300150) and Lx = 3:45 mm (0:6900150), Ly = 3:37 mm (0:67400150), respectively. Fig. 11 shows the simulated and measured return loss of the antenna arrays with the waveguide-to-SIW transition. The measured return losses follow the trend of the simulated ones well with a slight frequency shifting which may be caused by the fabrication tolerance and the permittivity fluctuation. For the arrays of WLR = 0:12 and 0.5, the measured impedance bandwidths are 3.3% and 6.5%, respectively, which are consistent with the simulated results. For the array with WLR = 0:71, the simulated impedance bandwidth reaches up to 11.5%, whereas the measured return loss exhibits a slight deterioration, namely 9 dB at 57.3 GHz and 8.2 dB at 59.2 GHz, respectively. The array with the larger WLR is more sensitive to the fabrication tolerance, because the slot with a bigger WLR is closer to the inductive window and the via-holes. In practical applications, the antenna can be directly integrated into circuits, and it can be expected that the impedance matching and reliability of the antenna without any transition would be improved.
ACKNOWLEDGMENT The authors would like to thank J. Xu, Y. Zhang, W. Liu, T. S. Ping See, and Z. Kuai for their valuable discussions and kind help in fabrication and measurement.
REFERENCES [1] X. Yu, D. Ni, S. Liu, Z. Li, and W. Wang, “Design of a wideband waveguide slot array antenna and its decoupling method for synthetic aperture radar,” in Proc. Eur. Microwave Conf., 2008, pp. 135–138. [2] W. Hong, N. Behdad, and K. Sarabandi, “Size reduction of cavitybacked slot antennas,” IEEE Trans. Antennas Propag., vol. 54, no. 5, pp. 1461–1466, May 2006. [3] J. Hirokawa, H. Arai, and N. Goto, “Cavity-backed wide slot antenna,” IEE Proc., vol. 136, no. 1, pp. 29–33, Feb. 1989. [4] Y. Miura, J. Hirokawa, M. Ando, Y. Shibuya, and G. Yoshida, “Double-layer full-corporate-feed hollow-waveguide slot array antenna in the 60 GHz-band,” IEEE Trans. Antennas Propag., vol. 59, no. 8, pp. 2488–2851, Aug. 2011. [5] G. Q. Luo, Z. F. Hu, L. X. Dong, and L. L. Sun, “Planar slot antenna backed by substrate integrated waveguide cavity,” IEEE Antennas Wireless Propag. Lett., vol. 7, pp. 236–239, 2008. [6] J. C. Bohorquez, H. A. F. Pedraza, I. C. H. Pinzon, J. A. Castiblanco, N. Pena, and H. F. Guarnizo, “Planar substrate integrated waveguide cavity-backed antenna,” IEEE Antennas Wireless Propag. Lett., vol. 8, pp. 1139–1142, 2009. [7] G. Q. Luo, Z. F. Hu, Y. Liang, L. Y. Yu, and L. L. Sun, “Development of low profile cavity backed crossed slot antennas for planar integration,” IEEE Trans. Antennas Propag., vol. 57, no. 10, pp. 2972–2979, Oct. 2009. [8] X. Chen, K. Wu, L. Han, and F. He, “Low-cost high gain planar antenna array for 60-GHz band applications,” IEEE Trans. Antennas Propag., vol. 58, no. 6, pp. 2126–2129, June 2010. [9] F. Xu and K. Wu, “Guided-wave and leakage characteristics of substrate integrated waveguide,” IEEE Trans. Microw. Theory Tech., vol. 53, no. 1, pp. 66–73, Jan. 2005. [10] A. S. Al-Zoubi, A. A. Kishk, and A. W. Glisson, “A linear rectangular dielectric resonator antenna array fed by dielectric image guide with low cross polarization,” IEEE Trans. Antennas Propag., vol. 58, no. 3, pp. 697–705, Mar. 2010. [11] T. Kai, J. Hirokawa, and M. Ando, “A stepped post-wall waveguide with aperture interface to standard waveguide,” in Proc. Antennas and Propagation Soc. Int. Symp., 2004, vol. 2, pp. 1527–1530.
Comments are closed.